Voltage reversal phenomenon is one of the main culprit of fuel cell failure!
Operation errors, harsh working conditions and the anode gas shortage caused by the external environment are the main culprits for the voltage reversal. The voltage reversal is mainly accompanied by the water electrolysis reaction and the carbon corrosion reaction, and the attenuation of the battery cannot be reversed. Optimizing system control strategies and developing anti-reverse electrode materials are the main countermeasures.
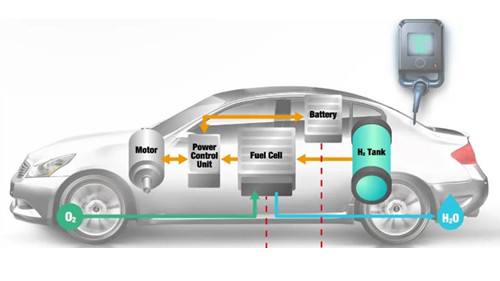
Cost, performance and durability are the three major obstacles to the commercialization of proton exchange membrane fuel cells. Among them, durability refers to the ability of a material or product to resist the long-term destructive effects of both itself and the objective environment. Generally, the working conditions that accelerate fuel cell degradation (affect durability) include start-stop, freezing/melting, idling (high potential), dry/wet cycle, and variable load. The partial and overall anode gas shortage caused by start-stop, load change, operation errors and external environment is an important factor that accelerates the attenuation of the fuel cell. At this time, the cell voltage is extremely low or even becomes negative, that is, reverse polarity occurs.
Under normal operating conditions, the oxygen reduction reaction (ORR) and the hydrogen oxidation reaction (HOR) occur at the cathode and anode of the fuel cell, respectively. The reaction formula is shown below. Among them EO is the standard electric potential difference, SHE is the standard hydrogen electrode.
When hydrogen shortage occurs at the anode, the anode needs an additional source of electrons and protons. Due to the “charge” effect, the anode voltage will increase until the voltage reverses (ie Eca-Ean<0 V), water electrolysis (oxygen precipitation) and carbon corrosion (carbon oxidation) occur. From a thermodynamic point of view, the carbon oxidation corrosion reaction is prone to occur when the anode potential is low (theoretically, see the following reaction formula), but the reaction kinetics is slow at this time and a higher potential (>1.0 V) is required. When the anode potential reaches 1.23 V or higher, the water electrolysis reaction becomes the main reaction. After a period of time, the water electrolysis reaction is deactivated and the carbon corrosion reaction is accelerated, which further increases the anode potential.
The reverse polarity of the voltage generates a lot of heat, which can cause pinholes in the proton membrane and cause a short circuit, eventually leading to catastrophic battery failure or accidents. At this time, one method is to optimize the system control strategy, such as continuous monitoring of voltage and exhaust, anode purging, etc., deliberately stopping or delaying the anode voltage rise, and the other is to use a new anti-reverse anode electrode (RTA). The anti-reverse anode electrode adds an oxygen evolution reaction catalyst to the catalytic layer or catalyst to make the water electrolysis effect greater than carbon corrosion. This method does not require voltage monitoring and can greatly improve the robustness of the stack. In order to solve the voltage reversal problem, most fuel cell vehicle developers optimize system control strategies, but the development of more durable and zero-defect fuel cells must be supported by material improvements.
From a material point of view, in order to deal with the problem of fuel cell voltage reversal, an oxygen evolution reaction electrocatalyst (OER), such as IrO2, RuO2, TiO2, and IrRu, is usually added in the preparation of the anode catalyst or catalytic layer. Due to the high cost of oxygen evolution catalysts, it is necessary to rationally design and develop anti-reverse fuel cells with oxygen evolution reaction catalysts to improve catalyst utilization. In order to understand the voltage reversal, the voltage reversal decay mechanism experiment is carried out here on the fuel cell with different oxygen evolution reaction catalyst loadings, focusing on the attenuation source, the decay process and the time scale of the reverse polarity.
The partial and overall undergassing phenomenon of anode caused by start-stop, load change, operation error and external environment can cause voltage reversal and pose great challenges to battery durability. As the anode voltage rises, the reverse electrode is usually accompanied by water electrolysis reaction and carbon corrosion reaction. In addition to protecting the battery from carbon corrosion through system control technology, the water electrolysis catalyst (IrO2) can actively protect the anode through oxygen evolution reaction (not carbon oxidation reaction) to increase the rate of proton and electron generation. Through testing, it can be found that the sustainable reverse polarity time of the membrane electrode containing the anti-reverse electrode catalyst is proportional to IrO2, and the electrodes with and without the addition of IrO2 catalyst experience water electrolysis and carbon corrosion in the reverse state. The degradation of battery performance caused by reverse polarity is mainly caused by the increase in ohmic resistance caused by the collapse of the anode structure. The imaging of the anti-reverse electrode catalyst electrode in the EOL stage found that no changes in the distribution and morphology of IrO2 particles were detected, indicating that the battery failure was caused by the catalytic deactivation of the oxygen evolution reaction catalyst. Regardless of whether the membrane electrode contains an IrO2 anti-reverse electrode catalyst, the membrane electrode exhibits a similar reverse-polarity decay behavior. The only difference is the reverse-polarity time.
For more news about fuel cells, please visit https://fuelcellcoating.blogspot.com/.
For detailed information on the preparation of fuel cell membrane electrode coatings, you can log on to Cheersonic’s official website https://www.cheersonic-liquid.cn/en/ultrasonic-spraying-for-battery/.